Introduction
Optical fiber lasers or fiber lasers for short belong to a group of solid-state lasers where the light is amplified in an optical fiber. The active material that provides the laser action is usually present in the fiber, the additive is usually a rare earth metal such as erbium (Er) or ytterbium (Yb). Lasers consisting of fiber-optic resonators and diode lasers are often included among fiber lasers (vide MOPA). The active medium is an optical fiber in fiber lasers, which can be 10 cm to a few hundred meters in length and a few µm to a few hundred µm in diameter. Due to their extremely high absorption and the length of gain, efficient pumping (see later) and high gain can be achieved. The maximum output power is restricted by the processes with losses due to small cross-section and large length and optical degradation. By integrating the various optical elements into the optical fiber, air conduction of the beam can even be completely eliminated and reliable, compact lasers can be built with high stability. In the early 1960s, shortly after the first laser was built the principle of laser amplification in a light guide fiber was devised and the first fiber laser was developed by Snitzer and his colleagues. Fiber laser technology started to evolve really fast from the mid-1980s. The main driving force was optical communication. In the last 10-15 years the main direction of development has been gaining increased power and short (ps-fs) pulse duration, which give scope for new areas of applications. High-power Yb-doped fiber lasers are more compact and more efficient, have more flexible beam guide and they are often a cheaper alternative in contrast to other type of lasers. Therefore they are more and more widely used for material processing (welding, cutting, drilling, milling of metals, plastics, ceramics, glass, etc.).
The basic operations of the laser
Lasers also undergo the process that happens with each generation of light. When elementary particles (atoms, molecules, electrons) have absorbed energy, they can move to a higher energy state and they are then referred to as being “excited.” Excited states are generally not stable; as elementary particles drop from higher-energy to lower-energy levels, they emit the extra energy as light. In addition, there is another phenomenon in lasers: light amplification created by forced photon emission (stimulated emission). The meaning of the acronym (LASER = Light Amplification by Stimulated Emission of Radiation) also describes this process: light amplification by stimulated emission of radiation.
An excited particle can drop its energy during light emission as follows: completely randomly, as is the case with conventional light sources (this is called spontaneous emission) or in the presence of an external effect (this is called stimulated emission). In stimulated emission an excited atom oscillate with the external field provided. One of the consequences of this oscillation is that it encourages electrons to decay to the lowest energy state. When this happens due to the presence of the electromagnetic field from a photon, a photon is released in the same phase and direction as the "stimulating" photon, so it amplifies the stimulating photon. Stimulated and spontaneous emission is present in each radiation process but usually spontaneous emission dominates. For the stimulated emission to be significant, many excited atoms must be created. It is required for laser operation that a state must be created where there are many excited atoms and few in the ground state (population inversion). The term inversion suggests that in atomic systems which are in temperature equilibrium with their environment, there are always more ground atoms than excited ones so laser operation requires the reversal (inversion) of this natural distribution. The medium in inversion state forms the material of the laser light amplifier (active medium). In some cases, it is sufficient in itself to produce powerful, coherent, low-divergence, monochromatic radiation, i.e., laser light. However, due to the losses, it is necessary to increase the efficiency of the amplification. In this case, feedback is required, where the photons pass through the active medium several times by inserting mirrors. Placing a pair of mirrors facing each other so that light emitted along the line between the mirrors is reflected back and forth increases intensity with each passing through the laser medium. If one of the mirrors is made with a reflectivity of less than 100%, some of the amplified light can be transmitted as a laser beam in each round. It is called optical output coupler. For a laser, continuous-wave operation implies that it is continuously pumped and continuously emits light. For a laser, pulsed operation implies that it lets the light pass only for a short period of time.
Laser beam characteristics
The unique properties of the laser beam make it useful and much more versatile in terms of applicability. The four most important properties are monochromaticity, spatial and temporal coherence, brightness, and directionality. Due to monochromaticity, i.e. self-coloured, lasers can be used at a given wavelength (unicolour light). The extent of beam spreading is characterized by directionality. This can have a minimum value. A beam with low divergence can be focused on a smaller spot. When we talk about the brightness of laser, which depends of their power, we have to make a distinction between continuous-wave laser and pulsed laser.
The laser beam can be continuous (CW) and pulsed. For pulsed lasers, knowledge of the pulse energy, pulse duration, pulse repetition rate and peak power are often required. Among continuous-wave lasers, the power of low-pressure gas lasers (e.g. He-Ne) often don’t achieve mW, however the power of high-pressure CO2 lasers and Nd: YAG lasers can be up to 10 kW. The peak power of pulsed lasers strongly depends on the pulse duration. Instantaneous power can be extremely high at the peak of a very short pulse. Lasers have generated peak power exceeding 1015 watts for intervals of about 10−12 second. High power and good focusing at the same time give high power density. If a 1 kW laser beam is focused on a 10 µm2 spot the power density could be 1014 W / m2. This value exceeds the surface of the Sun by six orders of magnitude.
The laser beam can be polarized but it is not necessary. However a significant number of applications require a polarized laser beam.
Optical fiber
Optical fibers are most often cylindrically symmetrical with the core. The operation of optical fibers is based on total reflection. In order for the phenomenon of total reflection to be occur the refractive index of the core must be greater than the refractive index of the cladding which surrounds the core. The function of the core is to transport the light, the role of the cladding is to prevent the exit of the light. A soft and solid coating can be applied to the sheath depending on the conditions and the application. The diameter of each part may vary depending on the application.
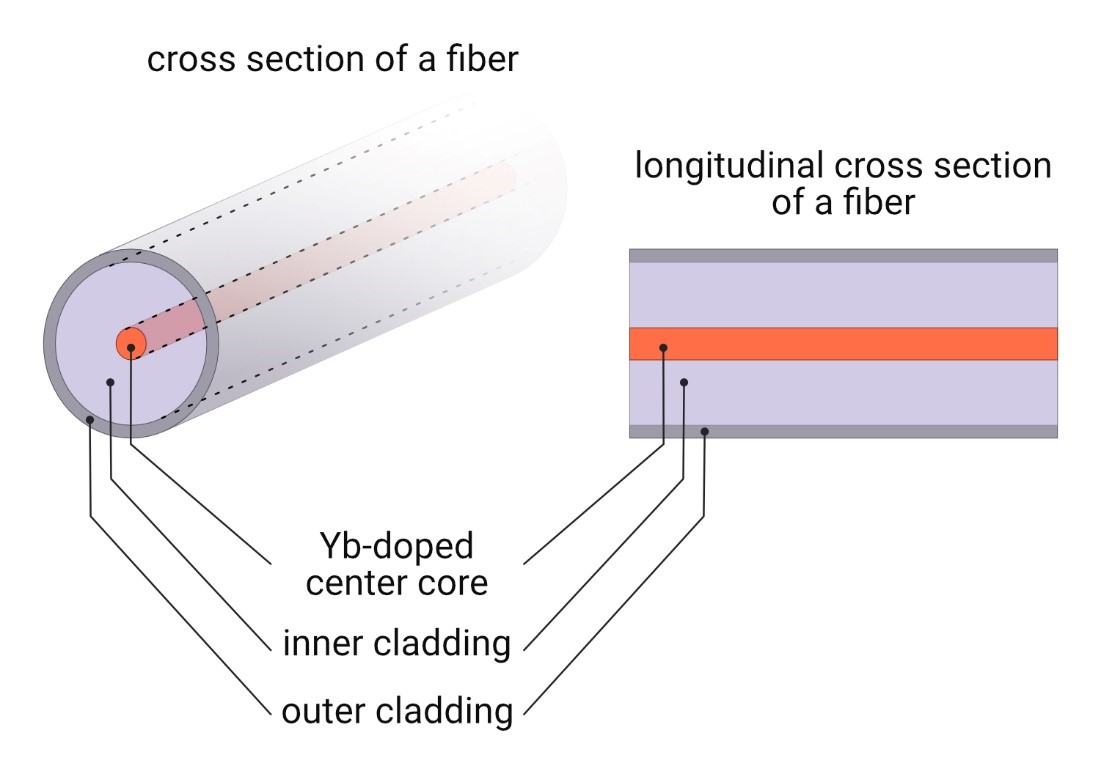
Bases of fiber laser
The structure of the fiber lasers is shown schematically in Figure 2. An optical fiber with a core containing an additive is the active material that amplifies. 2. ábra mutatja. Az aktív elem, ami az erősítést végzi egy adalékanyagot tartalmazó maggal rendelkező optikai szál.
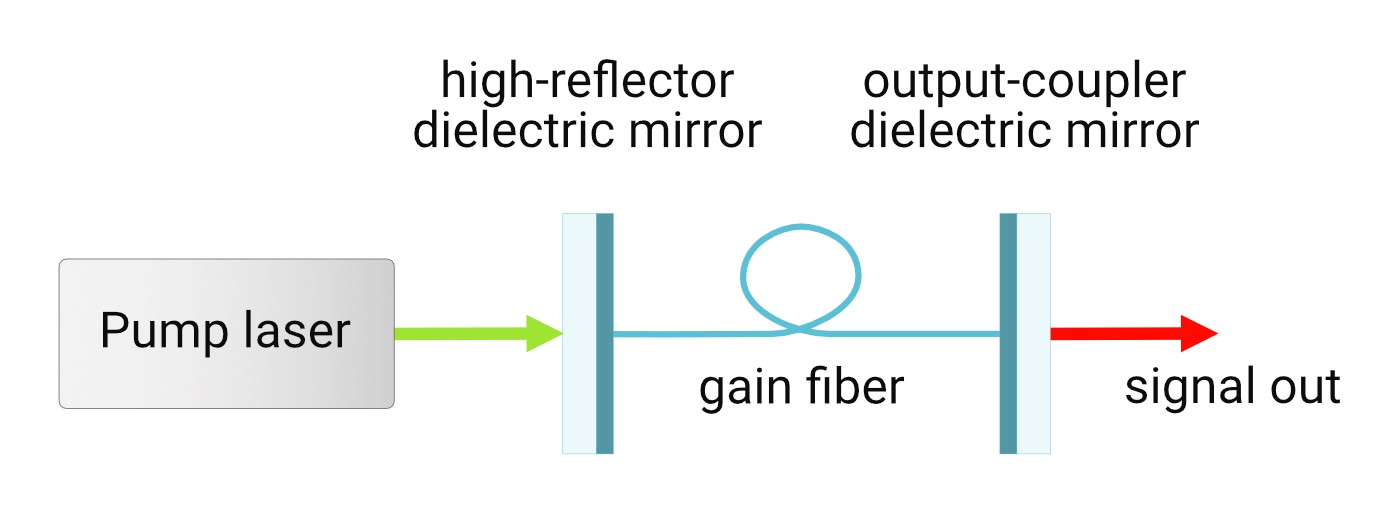
The core in the center of the fiber is doped with a rare-earth element, erbium (Er), neodymium (Nd), ytterbium (Yb), holmium (Ho), thulium (Tm), or praseodymium (Pr). The active material specifies the possible pumping and operating wavelengths and other parameters.
Doped element | Material of core | Laser wavelength (nm) | Pump wavelength (nm) |
Yb3+ | quartz glass | 1030-1100 | 900-980 |
Er3+ | quartz, phosphate - and fluoride glass | 1500-1600, 2700 | 1480, 1980 |
Nd3+ | quartz-,and phosphate glass | 1064-1088, 900-950, 1320-1350 | 808 |
Tm3+ | quartz-, germanium and fluoride glass | 1900-2100, 1450-1530, 480, 800 | 793 |
Pr3+ | quartz- and fluoride glass | 1300, 635, 600, 520 | 650 |
Ho3+ | quartz-and zirconia- fluoride glass | 2100, 2900 | 1064 |
Pumping is usually provided by one or more laser diodes. In the simplest case, the pumping light is launched into the core of the optical fiber that defer to numerical aperture. This process is provided by lens. The pumping light passes through a dichroic mirror that is permeable to pumping light but reflects the laser light. The mirror at the other end of the optical fiber is partially transmissive, and the laser beam is output coupled here. The dichroic and output coupling mirrors engineer the resonator of the laser. The output coupled laser beam is collimated by a lens so that the laser beam is refitted. The optical fiber is usually flexible to wind up, so that even longer resonator lengths can be placed in a very small space.
High-power fiber laser
The development of high-power fiber lasers up to 10 kW has been made possible by several technological factors. In particular, Yb doped fiber lasers are suitable for high power in continuous-wave and pulsed operation as well. The high-power laser diodes and the dual-cladding optical fibers are important factors along with the appropriate active material. This allowed cladding pumping which significantly increased the amount of gain, and thus the output power.
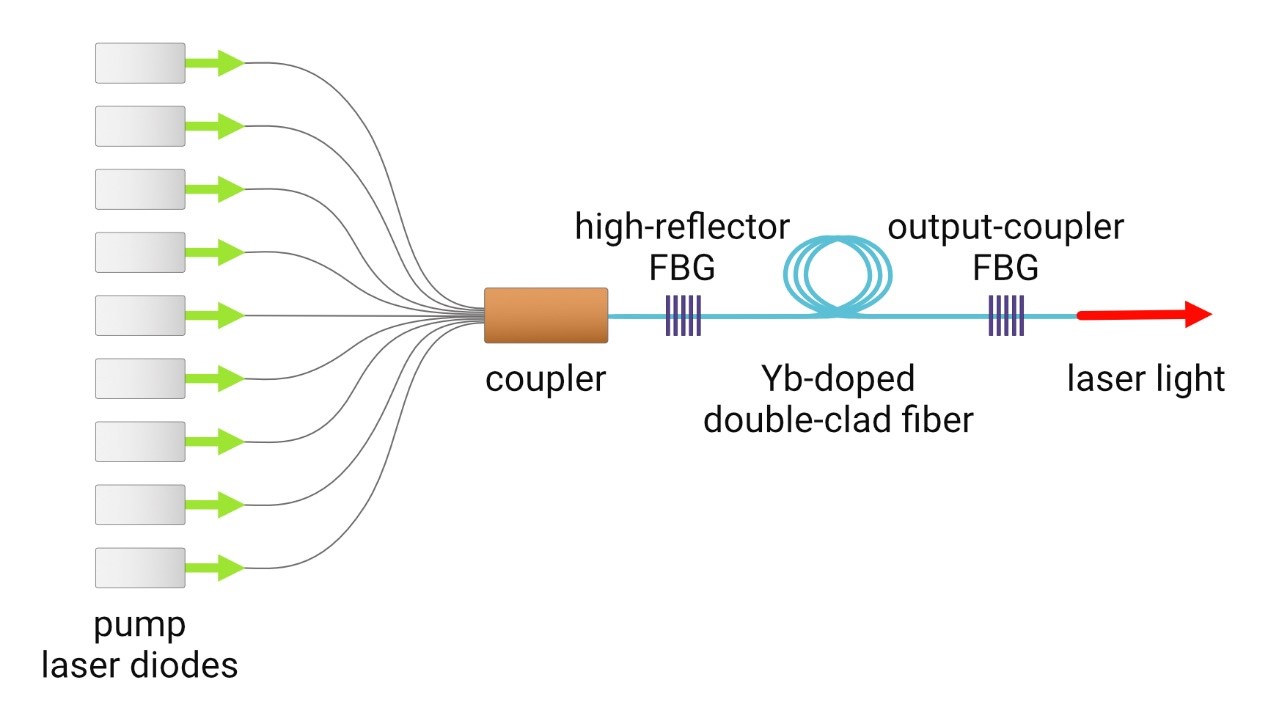
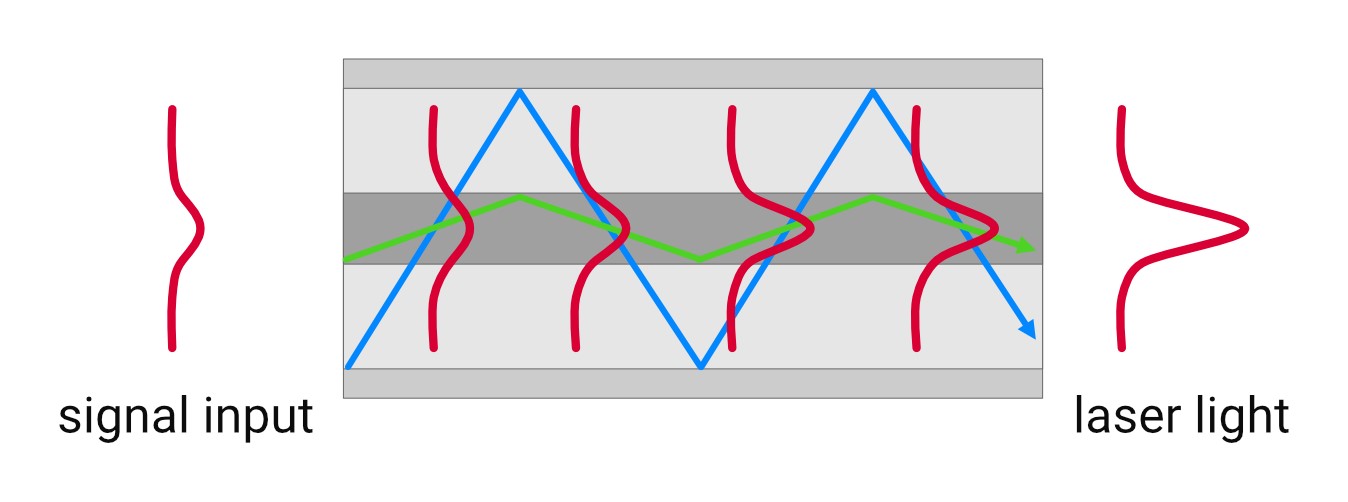
MOPA
A fiber MOPA system is recommended to achieve high-power fiber lasers. In this set-up, there is a seed laser that can be amplified in one or more circuits. The amplification is done in the optical fiber by coupling more laser diodes into the fiber with a combiner. This process is called pumping, and laser diodes are called pump diodes. The pump light from the pump combiner propagates through a double-clad fiber (active fiber) and the power is increased. If this process can be done more than once we are talking about more circuit amplification / pumping.
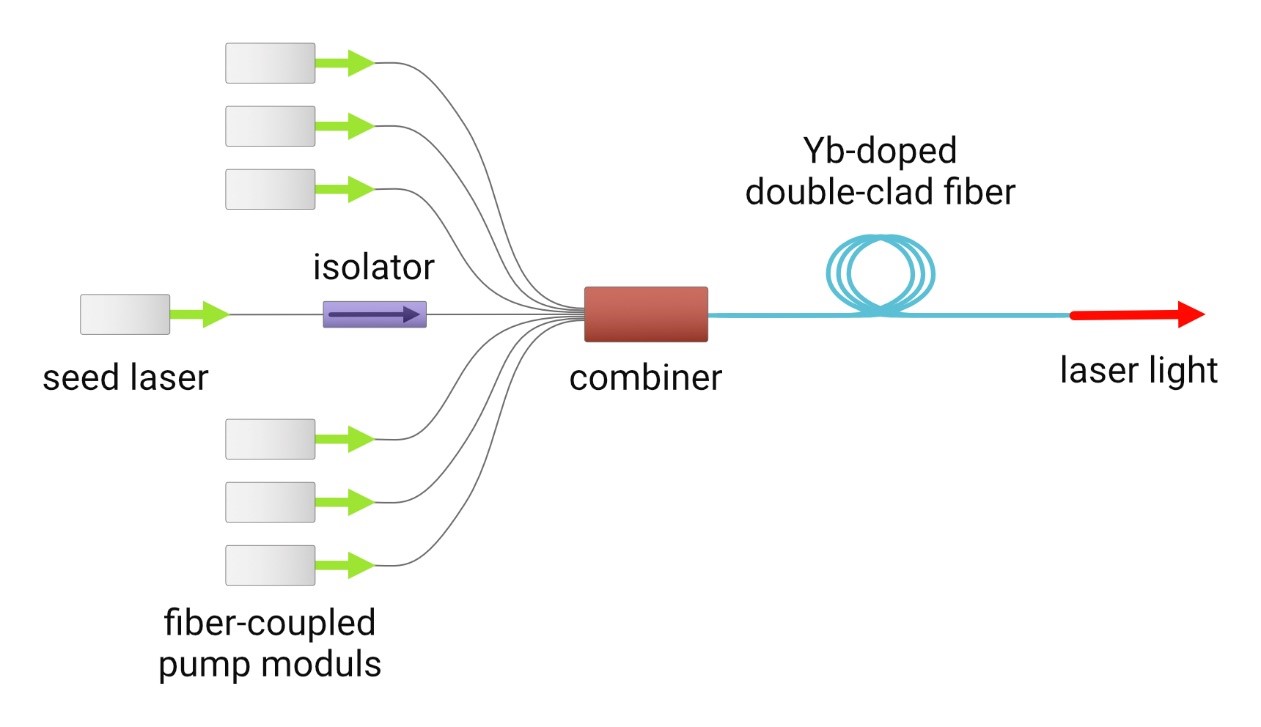
The choice of seed laser, the gain fiber, and the pump diodes depends on how much output power you wish and at what wavelength you would like to work. Apart from the above mentioned ones other optical devices are included in the system as well. The parameters of these depend on the desired end result.